POWER AMPLIFIERS PART TWO - HANDLING POWER
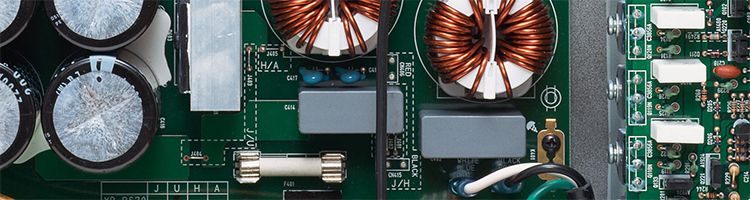
A power amplifier’s job is to supply a voltage to a loudspeaker and then to deliver whatever current the loudspeaker needs to move its voice coil, producing airwaves with a sound pressure level (SPL) up to what is specified for the speaker and/or application. The output voltage multiplied with the resulting current constitutes the power that is delivered to the loudspeaker.
If we connect a resistor - a ‘dummy load’ - to the amplifier’s output then things are simple; a resistor is a pure resistive and constant impedance. However, a loudspeaker is not a simple resistor, instead it can be seen as a coil with a resistance. This causes the loudspeaker’s ‘voltage to power’ transfer function to become complex, with the resulting impedance varying from the lowest ‘DC’ impedance to the impedance at the speaker’s resonance frequency, which can be much higher. The impedance listed in a loudspeaker’s data sheet is just an indication of the average.
More important is that a loudspeaker’s impedance is inductive, which means that voltage and current can be out of phase. Assuming we use a class AB amplifier, this poses a power issue. With a pure resistive load, at peak voltage the amplifier has to deliver also the peak current to the loudspeaker. The voltage inside the amplifier is the difference between the amplifier’s power supply voltage and the output voltage. So, when delivering a peak voltage to the loudspeaker, even at a very high current the power dissipated inside the amplifier is limited, as it’s the product of a high current and a low internal voltage difference.
With an inductive load, the phase between voltage and current changes with frequency. So there are frequencies where the current is out of phase with the voltage, asking the power amplifier to deliver the maximum current to the loudspeaker, while the voltage delivered to the loudspeaker is small. This means that the voltage difference inside the amplifier is much larger, compared to a pure resistive load. A high internal voltage difference multiplied by maximum current equals high power, so the power amplifier has to work much harder. This requires a lot from the output stage (the final power transistors and their cooling) and the power supply, especially with high power loudspeakers with low impedances,
Testing power amplifiers with real loudspeakers instead of resistive dummy loads is difficult; needing to use loudspeaker arrays in isolated rooms or the use of large and expensive inductive dummy loads. However, it is the only way to reveal how well the amplifier can handle high power ratings in real life situations.
The toughest thing to do to an amplifier is to play a continuous sine wave at maximum power. However, music is not a sine wave - it usually has some form of rhythm and is composed as a spectrum covering many frequencies. A good representation of the spectral and rhythmic property of ‘high energy’ music is pink noise, which has an average power of about 6dB less compared to the maximum power of a continuous sine wave. This ‘peak to average’ ratio is called the ‘crest factor’, and it is usually higher then 6dB for most music genres. Note that speakers peak power ratings are specified for short term, while a ‘noise’ or ‘music’ rating is published with a 6dB crest factor.
Apart from distortion - occurring when the power amplifier’s capabilities fall short of providing peak power - the main bottleneck is heat dissipation. As the power amplifier delivers power to the loudspeaker, heat builds up in the amplifier’s components, especially in the large output stage transistors. This is why these are often mounted on metal heat sinks, sometimes cooled by one or more fans.
Designing the power amplifier’s transistors, heat sinks and fans to cope with a continuous sine wave gives bulky, heavy and expensive enclosures. Most amplifiers, however, are designed for music, supporting continuous power assuming a certain crest factor, but still supporting full ‘peak power’ for a short duration. This is often done by a small microprocessor keeping track of the output power, protecting the circuits by limiting the output power whenever it exceeds a certain threshold for a longer time.